Department of Materials Science and Applied Chemistry
Research
Fields of Biotechnology & Materials Science
Biotechnology
Biotechnology is not simply a combination of biology and engineering but is an interdisciplinary field of study that fuses a variety of fields, including chemistry, physics, medicine, and biology. We research to understand the unique physical and chemical properties of nanomaterials and develop these into new medical technologies. Additionally, we research the effects of microorganisms and trace food ingredients in the environment to further understand how they affect humans.
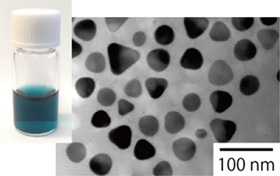
Dispersion and electron micrograph of silver nanoparticles that exhibit antibacterial activity
Analytical Chemistry
This field of research finds in what form something exists by using a variety of different methods. Technologies for isolating and analyzing target substances play an important role in a variety of fields, including all kinds of chemistry, biology, the environment, medicine, food, and more. We perform research mainly in biological and environmental analysis. Additionally, we use the findings we obtain to fabricate artificial biomolecules and attempt to use these to control biological functions.
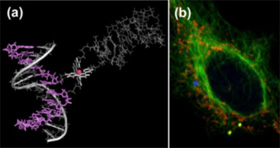
(a) An artificial nucleic acid that dynamically alters its structure in response to stimulation from a metal ion.(b) Live cell imaging of an mRNA granule with nucleic acid aptamers
Supramolecular Chemistry
Aligning atoms and molecules in regular patterns can express new functions not exhibited by the original atoms and molecules. Such collections of atoms and molecules are called supermolecules, and these supermolecules function in nature in even more complicated structures. Through creation of new supermolecules skillfully built up from simple molecules, we perform research for applications in a wide variety of fields, including optical materials, electronic devices, biomaterials, and medical materials.
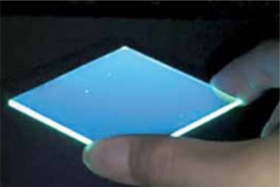
A supermolecule nanofiber-containing wavelength converting film intended to effectively utilize sunlight
Organic Materials
Organic materials refer to materials (compounds) constructed from oxygen, hydrogen, nitrogen, and other elements, with carbon as the main element. In our daily lives, these basic materials not only directly support our livelihoods in clothing (fabrics), plastics, rubber, wood, paper, fats, oils, surfactants, and foods, but also indirectly support cutting-edge technologies through their use in electronic information materials, like liquid crystals, organic light-emitting diodes, and organic semiconductors, as well as in medical materials like artificial organs, etc. Organic materials are beneficial in that they can be designed at the molecular level and can be freely selected for configuration or function, similar to the biological functions that occur in our own human bodies. These potential benefits and high degrees of freedom make organic materials very appealing to the desire of we as researchers to make progress. We are mainly involved in the development of light-related cutting-edge materials.
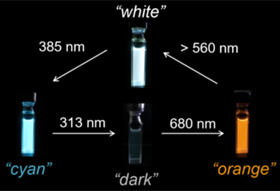
A new fluorescence switching molecular system that can selectively change the luminescent color depending on the wavelength of irradiating light.
Molecular Engineering
Substances that promote chemical reactions between molecules are called “catalysts,” and they play an important role in not just industrial chemical processes but also energy production and environmental protection. For example, catalysts are crucial in making fuel cells work, where hydrogen and oxygen chemically react to produce electricity and water; in making clean fuel from solar energy; and for cleaning automobile exhaust. We fundamentally design on the nano- and macro-level catalytic substances that contribute to the environment and energy, and we also perform applied research on these catalysts.
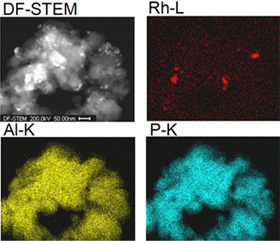
Electron micrograph and x-ray mapping of catalyst
Theoretical Computational Chemistry
This field of research makes advanced use of mathematical and physical theory, bioinformatics technologies, and computers to carefully examine the structure and function of substances, and design them and predict how they will behave. By using theoretical computational chemistry methods, we analyze a variety of substances, including catalytic and electrical materials that are important in the substance and environmental issues of medical materials, etc., related to vital phenomena. We recreate a nano-sized world on computers and investigate the structure of materials, the origin of their functionality, and their potential for use. We are also developing our own software for designing and discovering medical materials and new substances. We are putting special focus on technologies for exploring molecules.
Chemical Engineering
Chemical engineering is an important field of study and research that relates to a wide range of research fields, from analyzing reaction mechanisms at the molecular level to putting research into practice using full-scale equipment. In our laboratory, we perform various types of research into nanomaterial synthesis technologies, functional device development, supercritical fluid utilization technologies, biomass utilization technologies, energy-saving processes, natural compound recovery, etc., based around the keywords of “environment & energy,” “functional material synthesis processes,” “biomass,” “devices,” “recycling,” and “natural compounds.”
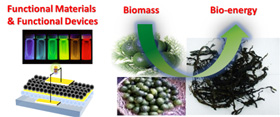
Functional material synthesis, device development, and biomass conversion
Polymer Materials
This field deals with the research of polymers. Polymers are enormous molecules that have a molecular mass of over 10,000. Polymers are deeply entwined with our daily lives, some examples being synthetic polymers found in plastics and fibers, inorganic polymers found in window glass and silicon rubber, and biopolymers such as proteins and DNA. Through the ideas of “creation (synthesis),” “investigation (structure),” and “utilization (function),” we perform research and development of polymer materials that possess unique properties.
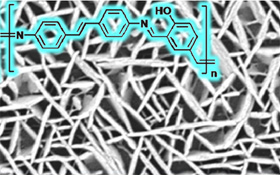
Nanowall structure in a polyazomethine π-conjugated polymer nano thin film
Inorganic Materials
This field of research aims to control the properties, shapes, sizes, etc., of inorganic compounds and develop materials that are useful to society. Inorganic materials, such as metallic oxides and carbon, play an important role in a variety of domains, including electronic components, catalysts, and building materials. We design, synthesize, and create devices from new inorganic materials, and perform research intended to discover the physicochemical and electrochemical properties and functions of these materials.
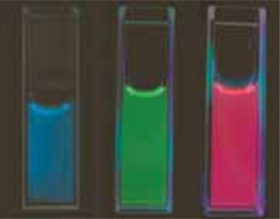
Oxide nanosheet luminescent material in 1 nm-thick sheets
Electrochemistry
This field of research involves oxidation-reduction reactions of materials, i.e., the electricity created from the giving and receiving of electrons between substances, as well as chemical energy conversion and material conversion. This field plays an important role in a variety of domains, including development of electricity storage devices, like batteries to decrease global carbon emissions, as well as in understanding vital phenomena such as transport of electrons in the respiratory chain. Using nanomeasurements of interfaces as our main method, we perform research into finding new nano structure formations that can help reduce the burden on the environment; new functions through catalyst design; developing electrochemical sensors; and elucidating electrode reaction mechanisms.
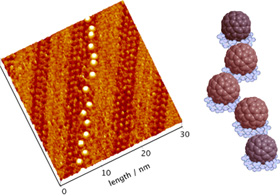
Nano-level visualizations of higher fullerenes and platinum porphyrin supermolecule structures
Enzyme Chemistry
Enzymes are proteins produced in the body that act as catalysts for chemical reactions in the body. Enzyme chemistry is the field of research that aims to understand chemically the functions of enzymes on the molecular level. Our main methods of research are exploring genes, which act as the blueprints of enzymes, and analyzing the specificity and speed of enzymatic reactions, and the molecular structure of enzymes. Through this research, we aim to develop detection and therapeutic drugs by searching for compounds that form the seeds of enzyme inhibitors and designing drugs to increase effects as enzyme inhibitors.
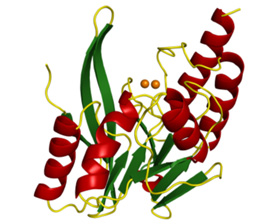
Molecular structure of metallo-β-lactamase, an enzyme produced by drug-resistant bacteria (the orange spheres are Zn(II) ions)
Materials Science and Engineering
Material Properties
In order to answer questions as to why metals are strong, how they can be made stronger, how they distort, and how they fail, we perform theoretical analyses via experiments and computer simulations on large monocrystals, small fine crystal grain materials, high purity materials, etc., and investigate metallic materials atom-by-atom on the nano-level to develop safer materials.
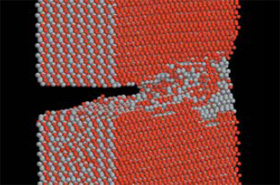
Atomic structure simulation of cracking period in a metallic material
Materials Structure Control
In order to build a safer, more reliable world, it is important to make invisible gases visible (to detect them). For example, sensing leaking gases can help prevent disasters, and detecting gases from human breath and skin can help in health management. For this, we control the shapes and structures of materials through hydrothermal treatments, electrochemical treatments, and chemical vapor deposition to develop devices on the micro- and nano-order. Additionally, we are able to find out new functions by assessing their properties.
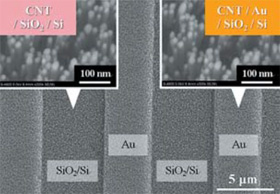
Devices with nano-sized gas adsorption antenna in close formation on a comb-shaped electrode (5 μm, 50 comb teeth)
Ecoprocessing
Many materials that support our everyday lives, including metals, ceramics, and semiconductors, are constantly evolving, and rapid progress in development of production methods is being made. Using previously unavailable techniques, like high magnetic and electric fields, we are attempting to produce high-function semiconductor thin films, anodized thin films, and microparticle zeolite.
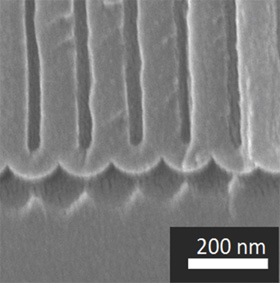
Aluminum anodized thin film produced in a 2 tesla magnetic field
Material Structure & Interface Control
Just like people, materials also have unique faces (microstructures). How these materials look also changes their personality (properties). By observing these materials at nano- and micro-scales using electron microscopes, etc., we can get an idea of the true face of a material and get hints about developing new materials. By analyzing, designing, and controlling these microstructures, we research and develop the basic materials that support the world, like materials for solar batteries and heat-resistant materials for turbines.
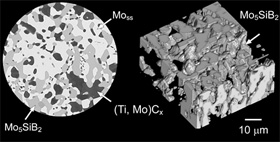
2D scanning electron microscope image and 3D image from the same perspective of the Mo5SiB2 layer only of an MoSiBTiC alloy we are developing as a super-high temperature material for use in next-generation jet engines
Advanced Materials
A material’s strength and function are controlled by the micro-scale microstructure that makes up the material, and in order to develop high performance materials, it is important to understand the properties and functions of the microstructure. By evaluation of mechanical properties of the world’s first minute, micron-sized specimens made from material microstructure, and by analysis of microstructure using a transmission electron microscope, we are designing and developing high-performance, high-function advanced materials.
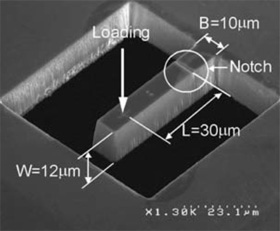
World’s smallest ultramicro notched bending test specimen. The specimen is smaller than 1/10th the width of a human hair, and we were the first in the world to successfully measure its fracture toughness and fatigue characteristics.
Harmonic Materials for the Environment
Environmental issues, most commonly exhibited in global warming, are becoming an ever more imminent threat to our livelihoods. In response to this, we wish to contribute to building a low-energy society by reducing the weight of metallic materials critical for transport equipment such as automobiles, trains, and airplanes. We are currently developing magnesium alloys with long period stacking ordered structure, which are very strong and both heat- and rust-resistant.
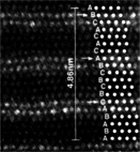
Transmission electron microscope image of high-strength magnesium alloy.
The white contrast points represent zinc and yttrium.
Through control of atomic level structure, we are able to develop materials with high strengths that surpass those of existing materials.
Functional Materials Design
We research and develop for the purpose of producing materials with superior functionality, focusing mainly on ceramic materials. We devise new material production processes and advanced microstructure control technologies while researching membrane material and materials for fuel cells that can be expected to be put to use in the environmental and energy fields. In membrane material research, we are researching materials called zeolites that have small pores on the atomic size order, and in fuel cell research, we are researching low-temperature operation solid oxide fuel cells, which are gaining attention as the next generation of fuel cells.
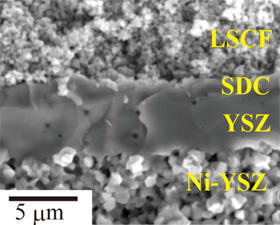
Scanning electron microscope image of high-performance solid oxide fuel cell consisting of multiple electrolyte layers
The layers labeled as YSZ and SDC are electrolytes, and those labeled Ni-YSZ and LSCF are electrodes
Carbon Nanomaterials
Carbon is a wonderful material that is light yet strong, exhibits a range of excellent properties, is environmentally friendly, and easily obtainable. By making nanomaterials like fullerenes and nanotubes out of carbon, there is the potential to make existing materials possess properties that were once unachievable. We develop original nanocarbon materials and are involved in solving problems in a wide variety of fields, including resources, the environment, energy, medicine, transport, and information.
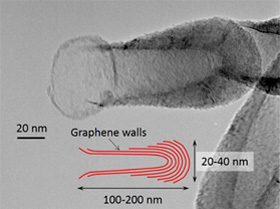
Transmission electron microscope image and structural schema of our newly developed “carbon nanopot.”
Microstructural Analysis
Structural control at the atomic- and nano-scales is extremely important to increase performance and function of substances and materials. In this laboratory, we perform nanostructural analysis using a transmission electron microscope with the aim of improving the functionality of functional materials like shape-memory alloys and structural materials like Ti base alloys, while researching how materials should be designed in order to produce new materials.
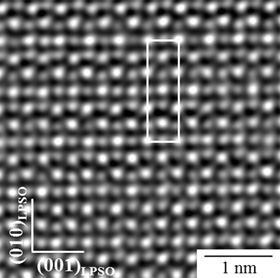
Transmission electron microscope of zirconium alloy that is a prospective high-temperature shape-memory alloy
Solid Mechanics
Ideal industrial products are high-performance and can be used safely for a long period of time. In order to create these, analytical methods for estimating with high precision the mechanical behavior of the constituent materials are necessary. In this laboratory, we mainly focus on constituent materials and develop assessable numerical methods for analysis of broad mechanical behavior based on microscope deformation mechanisms, while also performing research into applications in materials and structural design.
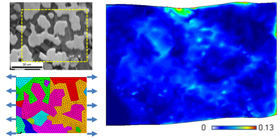
Results of KUMADAI Magnesium Alloy deformation analysis. We can quantitatively assess heterogenous deformations taking into account microstructure and single-crystal characteristics